Reference: November 2024 | Issue 11 | Vol 10 | Page 29
Bone formation and resorption are dynamic processes that exhibit diurnal variations. This circadian rhythm is regulated by food intake, and specifically, secretion and biological action of gut derived peptide hormones, including glucose-dependent insulinotropic polypeptide (GIP), glucagon-like peptide-1 (GLP-1), and glucagon-like peptide-2 (GLP-2). GIP and GLP-1 have well-established roles in regulating glucose and energy metabolism, whereas GLP-2 is better known for intestinotrophic actions.
However, in addition to this, these hormones can also influence bone turnover by directly, or indirectly, modulating the activity of osteoblast and osteoclast cells. As such, postprandial secretion of GIP, GLP-1, and GLP-2 inhibits bone resorption and/or promotes bone formation, representing a ‘gut-bone’ axis. Notably, GLP-1 and GIP/GLP-1 hybrid drugs are now clinically employed for diabetes and obesity, whereas GLP-2 drugs are used in the clinic to treat short bowel syndrome.
However, clinical data relating to the potential of these drugs to improve bone health is only now beginning to emerge. In addition, preclinical studies with dual-acting GIP/GLP-2 hybrid peptides demonstrate particular therapeutic promise for bone disorders, which merits further clinical exploration. This review summarises current literature on gut-derived hormones in relation to bone homeostasis, with particular emphasis on subsequent therapeutic application for bone disease.
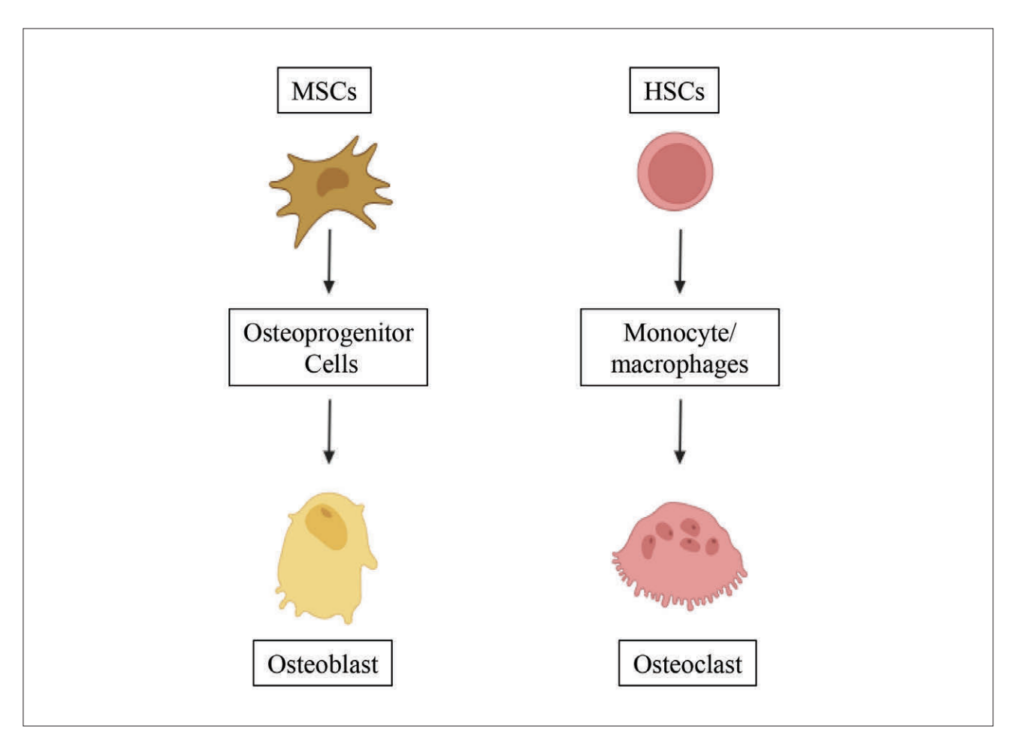
FIGURE 1: Origin of bone cells. Mesenchymal stem cells and haematopoietic stem cells represent precursors in the
development of osteoblasts and osteoclasts, respectively
Introduction
Bone is a dynamic tissue that serves as a structural framework for the body, facilitating movement, protecting vital organs, and acting as a reservoir for minerals such as calcium and phosphate in the form of hydroxyapatite.1 Along with the intestine and kidneys, bone plays a key role in regulating circulating calcium levels.1
The origin of bone tissue is rooted in early embryonic development, which follows two distinct pathways that include intramembranous ossification and endochondral ossification.1,2 Intramembranous ossification primarily occurs in the skull as well as a portion of the clavicle, where mesenchymal stem cells (MSCs) directly differentiate into osteoblasts (Figure 1). This process involves three main stages, namely proliferation, matrix maturation, and mineralisation, leading to the formation of bone without a prior cartilaginous phase.1,2,3
In contrast, endochondral ossification is the predominant mechanism controlling bone formation throughout the remainder of the skeletal system. It occurs in two main phases, firstly a cartilage template is formed in a non-vascularised environment, and then subsequently this template is gradually replaced by bone tissue through the formation of the periosteum, which harbours immature osteoprogenitor cells that differentiate into osteoblasts.
Osteoclasts are derived from haematopoietic stem cells (HSCs), specifically from the monocyte/macrophage lineage (Figure 1).1,2,3 These processes continue to play essential roles in bone growth, repair, and regeneration throughout life.
Disruption of the bone remodelling equilibrium leads to skeletal diseases including osteoporosis, Paget’s disease of bone, rheumatoid arthritis, as well as osteogenesis imperfecta.4 Further to this, metabolic disorders, for example diabetes and obesity, have been shown to negatively alter the bone microenvironment and compromise bone quality.5 A major component of this detrimental effect is thought to originate from obesity-diabetes induced changes in the secretion and biological action of gut-derived peptide hormones.
Thus, an emerging area of research into the relationship between gut health and bone metabolism, often referred to as the ‘gut-bone axis’, has gained much attention of late.5 For example, recent preclinical and clinical observations highlight the role of gut-derived peptides such as GIP, GLP-2, and to a lesser extent, GLP-1 in the maintenance of overall skeletal health (Figure 2),6,7,8 opening new avenues for the understanding and potential treatment of bone disorders.
In this review, the roles of GIP, GLP-1, and GLP-2 receptor signalling in the regulation of bone homeostasis are appraised (Figure 2). Mechanisms of action and implications for clinical practice are then considered, alongside the promise of continuing research to unlock new therapeutic opportunities for managing bone-related disorders and improving patient outcomes.
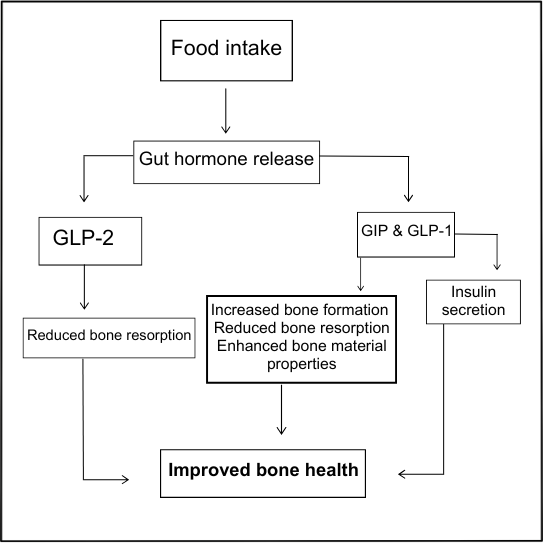
FIGURE 2: Schematic demonstrating the link between food intake, gut-derived hormones, and bone health. Emphasis is placed on secretion and action of GIP, GLP-1, and GLP-2 as part of the gut-bone axis. GIP and GLP-1 may exert direct and/or indirect positive effects on bone health through increased bone formation, reduced resorption, and improved bone material properties. For GLP-2, inhibition of bone resorption is mediated indirectly, as detailed within the text
Gut-bone axis
Overview
Bone remodelling exhibits well recognised diurnal variations, regulated by the circadian rhythm and influenced through the secretion of gut-derived peptide hormones.9 During the day, bone resorption is suppressed, presumably related to food intake and secretion of intestinal-derived hormones, whereas resorption increases at night and during periods of extended fasting (Figure 2).9,10
As well as gut hormones, bone remodelling is also known to be regulated by parathyroid hormone (PTH) and influenced by various other biochemical factors, such as calcitonin, oestrogen, thyroid hormones, glucocorticoids, insulin-like growth factors (IGFs), bone morphogenetic proteins (BMPs), prostaglandins, vitamin D, and transforming growth factor β (TGF-β),4,11 but the focus of this report will primarily be gut hormones.
Clinically, bone density can be assessed by dual-energy x-ray absorptiometry (DEXA), with interpretation used to diagnose or assess risk of osteoporosis.12 However, in addition to this, bone formation and resorption can be appraised through measurement of specific circulating biomarkers.
For bone formation, procollagen type 1 N propeptide (P1NP) and osteocalcin (OCN) serve as key indicators,13 both being secreted from osteoblasts. Bone resorption on the other hand, can be monitored through assessment of serum carboxy-terminal telopeptide of type I collagen (CTX-I) levels. In this regard, CTX-I, derived from mature type I collagen, directly reflects the degradation of collagen fibres during the process of bone resorption.7,8,13
Gut-derived peptides and bone
There is now unmistakable evidence to demonstrate that gut hormones play a key role in regulating bone metabolism, particularly through inhibition of bone resorption.10 Importantly, this suppression is not observed during daytime fasting when gut hormone secretion is reduced,14 highlighting direct effects of peptide hormones on bone turnover in response to nutritional status.
Moreover, reductions of circulating CTX-I are more pronounced following oral glucose administration than intravenous glucose infusion, further indicating that gut derived hormones, rather than glucose levels per se, modulate bone turnover.15 As such, GIP, secreted by enteroendocrine K-cells, and GLP-1, released from corresponding intestinal L-cells, collectively referred to as the incretin hormones, are well-studied for their roles in glucose homeostasis and energy balance.6,7,8,9,10,16
Indeed, GLP-1 receptor (GLP-1R) agonists, such as liraglutide and semaglutide, are approved for the treatment of both type 2 diabetes (T2D) and obesity.7,8,17 Moreover, the dual GIP and GLP-1 receptor agonist tirzepatide has also recently been clinically approved for the same applications.18 GLP-2, also secreted by enteroendocrine L-cells, differs from GLP-1 and GIP in that it appears to have less influence on metabolic control and primarily functions as an intestinotrophic factor, helping maintain intestinal health.3,19
On this basis, the long-acting GLP-2 mimetic, teduglutide, has gained clinical approval for short bowel syndrome.20 Thus, drugs utilising GIP, GLP-1, or GLP-2 receptor signalling pathways are already in clinical use, which should make potential application within the bone disease arena less complicated (Figure 3).
Diabetes, obesity, and bone health
In conditions such as obesity and T2D, the balance between bone formation and resorption is disturbed, leading to bone health issues.5 There is no doubt that chronic hyperglycaemia, a hallmark of all types of diabetes, as well as insulin resistance, adversely affects bone integrity.5,6 In addition, receptor activator of nuclear factor kappa beta ligand (RANKL), that plays a crucial role in osteoclast activity and bone resorption,21 also directly influences pancreatic beta-cell function and overall metabolism, with its activity being compromised in obesity and T2D.5,21
Furthermore, 24 months treatment with the anti-osteoporotic drugs denosumab (Dmab) and alendronate in T2D lead to improvements of bone mineral density (BMD), but Dmab also induced a modest improvement in glycaemic control when compared to alendronate.22 This further highlights the link between glucose regulation, T2D, and bone disease, suggesting Dmab may offer dual benefits in managing both bone health and hyperglycaemia.
Hyperglycaemia can also elevate levels of tumoir necrosis factor (TNF)-α and interleukin (IL)-6, cytokines linked to increased osteoclast activity.5,6 Additionally, high glucose levels negatively impact osteoblast function, contributing to bone defects partly through the production of advanced glycation end-products (AGEs), which upregulates sclerostin, an inhibitor of osteoblast activity.23 Studies in insulin-resistant and insulin-deficient animal models, as well as humans, continue to highlight the adverse effects of obesity and T2D on bone integrity.5-8,10
Ultimately, obesity and T2D are associated with detrimental structural changes in bone, such as decreased trabecular bone connectivity and cortical bone thickness, increasing the risk of fractures and impairing healing.5-8 In this regard, it is well recognised that the secretion and action of GIP and GLP-1 is disturbed in obesity and T2D,5-10,16-18 which could also directly impact skeletal health. It follows that drugs used for the treatment of T2D, and especially those that increase bioactivity of gut-derived hormones, could exert added benefits on bone health.11,15-18
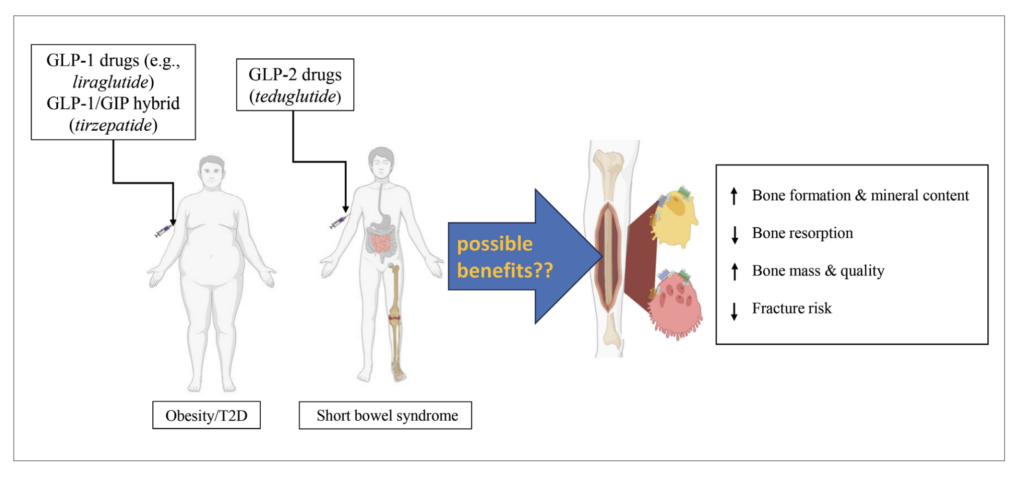
FIGURE 3: Potential impact of clinically approved GLP-1, GLP-2 as well as GIP/GLP-1 hybrid peptides on bone. GLP-1 drugs (exenatide, liraglutide etc), as well as GIP/GLP-1 hybrid peptides (tirzepatide), are clinically approved for diabetes and/or obesity, with GLP-2 based drugs (teduglutide) approved for the treatment of short bowel syndrome. Potential additional beneficial effects of these drugs on bone are depicted
GLP-1
GLP-1 plays a key role in maintaining glucose homeostasis by enhancing glucose-dependent insulin secretion from pancreatic beta-cells,7 as well as inhibiting glucagon secretion and suppressing appetite.7,10 The mechanisms by which GLP-1 influences bone remodelling are thought to involve several pathways. GLP-1R activation in osteoclasts can modulate bone turnover through the mitogen-activated protein kinase (MAPK) and nuclear factor kappa B (NF-κB)/MAPK- nuclear factor of activated T-cells 1 (NFATc1) pathways, respectively.24 However, the GLP-1R is not evidenced in mature osteoblasts, but it is expressed in bone marrow stromal cells (BMSCs). Furthermore, GLP-1 has been shown to stimulate calcitonin secretion from the thyroid gland, which may further contribute to protective effects on bone,25 but whether this action is relevant in humans still needs to be clarified.26
GLP-1 and effects on bone metabolism
Several studies show that the GLP-1R is expressed in some bone cells, including osteoclasts and BMSCs, but appears to be absent in mature osteoblasts, indicating a complex role in bone homeostasis that is incompletely understood.27 In vitro activation of the GLP-1R by exendin-4 enhanced osteogenic differentiation while inhibiting adipogenic differentiation of BMSCs.28 In addition, liraglutide enhanced osteoblastic differentiation and proliferation in MC3T3-E1 cells by activating ERK5 signalling pathway.28
In rodent models, genetic knockout of the GLP-1R reduces bone quality and maturity of the collagen matrix.29 In keeping with this, the GLP-1 mimetics exendin-4 and liraglutide improve trabecular bone microarchitecture and reduce osteoclast activity in ovariectomised (OVX) mice.30 Additionally, GLP-1 and exendin-4 restored femur and vertebrae bone mass in hyperlipidaemic and hypercaloric Wistar rats.31 In OVX insulin-deficient streptozotocin (STZ) diabetic rats, liraglutide significantly lowered serum CTX-I levels and inhibited osteoclast differentiation.32 Fortunately, successful introduction of GLP-1 drugs into clinical practice has facilitated more detailed study of GLP-1 effects on bone health in the human setting (Figure 3).
Accordingly, liraglutide was initially found to increase bone formation and preserve bone mineral content in obese women, providing early clinical evidence of GLP-1 drug benefits on bone health.33 Moreover, liraglutide treatment for 26 weeks reduced body weight without adversely affecting bone mineral density (BMD) in patients with T2D.34 Equally, the GLP-1 mimetics exenatide, dulaglutide, liraglutide, albiglutide, and semaglutide helped preserve bone density and reduce fracture risk in older adults with T2D.35
Notably, this protective effect appears to be dependent on the duration of treatment, with only a GLP-1RA treatment period exceeding 52 weeks being associated with decreased fracture risk.36 In good accordance, increased postprandial GLP-1 levels are associated with a reduced risk of osteoporosis in non-diabetic postmenopausal women.37 In addition, Zhang et al (2020) found that a single nucleotide polymorphism (SNP) in GLP-1R, rs2295006, was negatively associated with lumbar and total hip BMD in postmenopausal women, and similar observations have been made more recently by others.38,39 That said, there are also reports of no significant impact of exenatide on BMD or fracture risk in T2D,40 with subsequent meta-analyses also yielding conflicting outcomes.41,42
Overall, whilst slightly inconsistent, there does appear to be a neutral or potential positive impact of GLP-1R signalling on bone health. Furthermore, the impact of GLP-1 mimetics to inhibit appetite and reduce body weight should also be considered when interpreting effects of these drug on bone turnover in humans.
GIP
Similar to GLP-1, GIP is best known as an incretin hormone with fundamental effects on the maintenance of glucose homeostasis.16,18 However, in addition to this, the presence of functional GIP receptor (GIPR) on bone osteoblast cells has been confirmed many years ago.43 Similarly, Zhong et al (2007) identified GIPRs on murine osteoclasts, suggesting an important and direct impact of GIP in both the formation and breakdown of bone tissue.44
GIP and effects on bone metabolism
In agreement with direct receptor effects on osteoblasts and osteoclasts, GIP exerts both an anabolic and anti-catabolic action in bone.10,16,18 Initial observations in rodents demonstrated that native GIP positively affected bone density in OVX rats.43 Subsequent preclinical research utilising enzyme-resistant long-acting GIP peptides, as well as genetic manipulation of the GIPR, reinforced these early findings. For instance, in STZ-diabetic mice the GIPR agonist, (D-Ala2)GIP, improved bone quality and halted matrix degradation, ultimately enhancing fracture resistance.45
Additional studies confirmed that N-AcGIP, another long-acting GIP agonist,46 decreased osteoclast-mediated bone resorption and enhanced cortical bone quality in rodents.46 In keeping with this, transgenic mice overexpressing GIP exhibit increased bone mass and enhanced osteoblast activity at 24 months of age, whilst GIPR KO leads to decreased BMD, cortical thickness, and overall bone strength.47,48
Human studies yield somewhat mixed results regarding the impact of GIP on bone metabolism. Early studies found no significant effect of intravenous GIP on bone resorption,10 whereas more recently, GIP infusion was shown to suppress the bone resorption marker CTX-I and transiently increase the bone formation marker P1NP in people with T1D as well as healthy controls.16,49 Similarly, Skov-Jeppesen et al (2019) reported that subcutaneous GIP injection increased P1NP by 15 per cent in healthy men, that is thought to be associated with transiently lowered PTH levels.50,51
Moreover, GIP infusion significantly reduced CTX-I levels in healthy humans,52 as well as in T1D or T2D,53 indicating a robust suppression of bone resorption in various settings that is independent of the established effects of GIP on insulin secretion. Further investigations of inherent variations of the GIPR within the normal population reveal that the missense GIPR variant, rs1800437 (Glu354Gln) that results in diminished GIPR signalling, leads to lower BMD and increased risk of non-vertebral fractures in perimenopausal Danish women.54
Interestingly, in a similar study of 426,824 individuals, another GIPR variant, Glu288Gly, was associated with lower BMD, but no increase in fracture risk.55 Indeed, a more recent large meta-analysis confirmed that while GIPR variants may influence BMD, this does not necessarily translate to increased bone fracture risk.56 Taken together, it is clear that GIPR signalling affects bone turnover, but the overall significance of these effects need to be fully determined. Interestingly, tirzepatide, a dual-acting GIP/GLP-1 receptor agonist that is now clinically approved for both obesity and T2D, was designed with an amino acid sequence closely resembling that of native GIP, resulting in strong preference for GIPR binding over the GLP-1R.
This unique receptor activity makes tirzepatide a promising candidate for improving bone health in obesity and diabetes, through the positive effects of GIP, and potentially GLP-1, on bone metabolism. Although tirzepatide has not demonstrated anabolic bone actions in animals,57 an assessment report from the European Medicine Agency observed trends of decreased P1NP and increased CTX-I levels in people with T2D after 29 days of treatment. The exact mechanism behind this action on bone remains unclear, but likely relates to benefits of GIP, and perhaps GLP-1, on bone as described in (Figure 3).58
GLP-2
The primary physiological effect of GLP-2 appears to be within the intestine, where GLP-2 promotes small and large intestinal growth through stimulating cell proliferation and inhibiting apoptosis in the crypt compartment.19 GLP-2 also enhances intestinal barrier function, increases glucose transport, and boosts mesenteric blood flow.50 Together this has led to the approval of long-acting GLP-2 drugs for the treatment of short bowel syndrome.20 In relation to bone, GLP-2 receptors have been detected in osteoblast-like cell lines such as MG-63 and TE-85,59 but presence in mature osteoblasts and other bone-related cells is yet to be confirmed.
This has led to speculation that GLP-2 may act indirectly on bone cells in humans, with the presence of GLP-2R on the parathyroid gland suggesting a potential link between GLP-2, PTH secretion, and bone turnover. Consistent with this, the anti-resorptive effect of GLP-2 is absent in patients with hypoparathyroidism,50 further supporting a role of PTH in GLP-2 mediated effects on bone in humans.
GLP-2 and effects on bone metabolism
In osteoporotic (SAMP6) mice, six-week treatment with GLP-2 was shown to reduce bone loss and improve bone microstructure.60 In contrast, the long-acting enzyme resistant form of GLP-2, namely (Gly2)GLP-2, did not provide benefit on bone strength in a mouse model of bone fragility,61 despite promoting the expression of bone matrix genes and reducing osteoclast number in the in vitro setting.60 However, in humans, early studies demonstrated that GLP-2 administration for five weeks increased spinal BMD in people with short bowel syndrome,62 but had limited overall bone benefits.
Interestingly, GLP-2 administration leads to a reduction in PTH levels in people with an intact intestine, but not in those with short bowel syndrome,63 indicating that an intact small intestine is required for GLP-2 mediated suppression of PTH secretion. Further to this, a dose escalation study with GLP-2 (100, 200, 400, and 800µg, single subcutaneous injections) in postmenopausal women revealed dose-dependent reductions of CTX-I levels and related bone resorption,10 that are believed to be sustainable in nature.64 Overall, GLP-2 appears to be an important regulator of bone metabolism, primarily through inhibiting bone resorption, but with minimal effects on bone formation.
Clinical relevance and future studies
The relationship between diabetes, obesity, and bone health is of significant clinical importance. Obesity and T2D disrupt the balance between bone formation and resorption, leading to increased bone fragility and fracture risk.65 In this regard, the incretin hormones, namely GIP and GLP-1, represent key targets for the treatment of T2D and obesity, but are also known to play significant roles in bone metabolism, especially in terms of GIP. In addition, GLP-2, an intestinotrophic hormone with clinical application for short bowel syndrome, reduces bone resorption. Thus, drugs that target GIP, GLP-1, or GLP-2 receptor signalling pathways could be employed for treating bone diseases.
The potential success of this approach is enhanced given that GIP, GLP-1, and GLP-2 based drugs are already in clinical use, although postulated benefits on bone health still need to be fully confirmed (Figure 3). Thus, whilst data linked to specific bone effects of these clinically approved drugs in humans is still evolving, the initial outlook is positive. In addition, multi-acting unimolecular peptides have emerged as highly promising therapeutics in obesity and T2D, typified by the GIP/GLP-1 hybrid peptide tirzepatide.
Observations relating to the impact of tirzepatide on bone are still very much in their infancy, but there are suggestions that this drug could improve bone strength and reduce fracture risk. Further to this, early preclinical work with dual GIP/GLP-2 hybrid analogues demonstrates considerable therapeutic promise. Specifically, GL-0001 represents a first-in-class dual-acting GIP/GLP-2 peptide that improves bone biomechanical properties, preserves trabecular bone structure as well as increasing collagen cross-linking.66 Targeting multiple peptide hormone receptors with the same drug entity appears to be the most promising strategy, where additive, or even synergistic, benefits of simultaneously activating two receptor signalling pathways in bone can be fully realised.
Conclusion
Gut hormones play a fundamental role in controlling bone homeostasis and maintaining optimal bone strength and quality. Preclinical studies in rodents with long-acting GIP, GLP-1, and GLP-2 analogues, alongside related clinical trials, suggest these molecules are safe, and furthermore, may improve overall bone health. Additionally, agents capable of positively modulating more than one peptide hormone receptor simultaneously, such as tirzepatide or the recently characterised GL-0001 peptide, could offer an even more exciting avenue to help realise the potential of gut-derived hormones to treat bone disease.
Acknowledgements and funding
The authors’ work on gut-derived hormones and obesity-diabetes has been generously supported over many years by Diabetes UK, Invest Northern Ireland, the Irish Endocrine Society, Northern Ireland Department for the Economy, and Ulster University Strategic Funding.
References
- Su N, Yang J, Xie Y, et al. Bone function, dysfunction and its role in diseases including critical illness. Int J Biol Sci. 2019;15(4):776-787.
- Olsen BR, Reginato AM, Wang W. Bone development. Annu Rev Cell Dev Biol. 2000;16:191-220.
- Percival CJ, Richtsmeier JT. Angiogenesis and intramembranous osteogenesis. Dev Dyn. 2013;242(8):909-22.
- Feng X, McDonald JM. Disorders of bone remodeling. Annu Rev Pathol. 2011;6:121-45.
- Wang J, You W, Jing Z, et al. Increased risk of vertebral fracture in patients with diabetes: A meta-analysis of cohort studies. Int Orthop. 2016;40(6):1299-307.
- Ali A, Flatt PR, Irwin N. Gut-derived peptide hormone analogues and potential treatment of bone disorders in obesity and diabetes mellitus. Clin Med Insights Endocrinol Diabetes. 2024;17:11795514241238059.
- Mabilleau G, Pereira M, Chenu C. Novel skeletal effects of glucagon-like peptide-1 (GLP-1) receptor agonists. J Endocrinol. 2018;236(1):R29-R42.
- Mabilleau G, Bouvard B. Gut hormone analogues and skeletal health in diabetes and obesity: Evidence from preclinical models. Peptides. 2024; 177:171228.
- Bjarnason NH, Henriksen EE, Alexandersen P, et al. Mechanism of circadian variation in bone resorption. Bone. 2002;30(1):307-13.
- Henriksen DB, Alexandersen P, Bjarnason NH, et al. Role of gastrointestinal hormones in postprandial reduction of bone resorption. J Bone Miner Res. 2003;18(12):2180-9.
- Chen YJ, Jia LH, Han TH, et al. Osteoporosis treatment: Current drugs and future developments. Front Pharmacol. 2024;15:1456796.
- Blake GM, Fogelman I. The role of DXA bone density scans in the diagnosis and treatment of osteoporosis. Postgrad Med J. 2007;83(982):509-17.
- Kuo TR, Chen CH. Bone biomarker for the clinical assessment of osteoporosis: Recent developments and future perspectives. Biomark Res. 2017;5:18.
- Kueper J, Beyth S, Liebergall M, Kaplan L, Schroeder JE. Evidence for the adverse effect of starvation on bone quality: A review of the literature. Int J Endocrinol. 2015;2015:628740.
- Lei WS, Kilberg MJ, Zemel BS, et al. Bone metabolism and incretin hormones following glucose ingestion in young adults with pancreatic insufficient cystic fibrosis. J Clin Transl Endocrinol. 2022;30:100304.
- Bergmann NC, Lund A, Gasbjerg LS, et al. Separate and combined effects of GIP and GLP-1 infusions on bone metabolism in overweight men without diabetes. J Clin Endocrinol Metab. 2019 Jul 1;104(7):2953-2960.
- Nauck MA, Quast DR, Wefers J, Meier JJ. GLP-1 receptor agonists in the treatment of type 2 diabetes – state-of-the-art. Mol Metab. 2021;46:101102.
- Nauck MA, D’Alessio DA. Tirzepatide, a dual GIP/GLP-1 receptor co-agonist for the treatment of type 2 diabetes with unmatched effectiveness regrading glycaemic control and body weight reduction. Cardiovasc Diabetol. 2022;21(1):169.
- Drucker DJ, Erlich P, Asa SL, Brubaker PL. Induction of intestinal epithelial proliferation by glucagon-like peptide 2. Proc Natl Acad Sci US. 1996;93(15):7911-6.
- Jeppesen PB, Lund P, Gottschalck IB, et al. Short bowel patients treated for two years with glucagon-like peptide 2: Effects on intestinal morphology and absorption, renal function, bone and body composition, and muscle function. Gastroenterol Res Pract. 2009;2009:616054.
- Vega D, Maalouf NM, Sakhaee K. CLINICAL Review: The role of receptor activator of nuclear factor-kappaB (RANK)/RANK ligand/osteoprotegerin: Clinical implications. J Clin Endocrinol Metab. 2007;92(12):4514-21.
- Wang K, Gao L, Liu C, Bao X, Tian Y, Li Y. Denosumab improves glycaemic parameters in postmenopausal osteoporosis patients with combined type 2 diabetes mellitus. Cell Mol Biol. 2023;69(8):185-191.
- Piccoli A, Cannata F, Strollo R, et al. Sclerostin regulation, microarchitecture, and advanced glycation end-products in the bone of elderly women with type 2 diabetes. J Bone Miner Res. 2020;35(12):2415-2422.
- Li Z, Li S, Wang N, Xue P, Li Y. Liraglutide, a glucagon-like peptide-1 receptor agonist, suppresses osteoclastogenesis through the inhibition of NF-κB and MAPK pathways via GLP-1R. Biomed Pharmacother. 2020;130:110523.
- Yamada C, Yamada Y, Tsukiyama K, et al. The murine glucagon-like peptide-1 receptor is essential for control of bone resorption. Endocrinology. 2008;149(2):574-9.
- Bjerre Knudsen L, Madsen LW, Andersen S, et al. Glucagon-like Peptide-1 receptor agonists activate rodent thyroid C-cells causing calcitonin release and C-cell proliferation. Endocrinology. 2010;151(4):1473-86.
- Meng J, Ma X, Wang N. Activation of GLP-1 receptor promotes bone marrow stromal cell osteogenic differentiation through β-Catenin. Stem Cell Reports. 2016;6(4):579-591.
- Sun Y, Liang Y, Li Z, Xia N. Liraglutide promotes osteoblastic differentiation in MC3T3-E1 cells by ERK5 pathway. Int J Endocrinol. 2020;2020:8821077.
- Mabilleau G, Mieczkowska A, Irwin N, Flatt PR, Chappard D. Optimal bone mechanical and material properties require a functional glucagon-like peptide-1 receptor. J Endocrinol. 2013;219(1):59-68.
- Pereira M, Jeyabalan J, Jørgensen CS, et al. Chronic administration of Glucagon-like peptide-1 receptor agonists improves trabecular bone mass and architecture in ovariectomised mice. Bone. 2015;81:459-467.
- Nuche-Berenguer B, Lozano D, Gutiérrez-Rojas I, et al. GLP-1 and exendin-4 can reverse hyperlipidic-related osteopaenia. J Endocrinol. 2011;209(2):203-10.
- Wen B, Zhao L, Zhao H, Wang X. Liraglutide exerts a bone-protective effect in ovariectomised rats with streptozotocin-induced diabetes by inhibiting osteoclastogenesis. Exp Ther Med. 2018;15(6):5077-5083.
- Iepsen EW, Lundgren JR, Hartmann B, et al. GLP-1 receptor agonist treatment increases bone formation and prevents bone loss in weight-reduced obese women. J Clin Endocrinol Metab. 2015;100(8):2909-17.
- Hygum K, Harsløf T, Jørgensen NR, Rungby J, Pedersen SB, Langdahl BL. Bone resorption is unchanged by liraglutide in type 2 diabetes patients: A randomised controlled trial. Bone. 2020;132:115197.
- Zhang YS, Weng WY, Xie BC, et al. Glucagon-like peptide-1 receptor agonists and fracture risk: A network meta-analysis of randomised clinical trials. Osteoporos Int. 2018;29(12):2639-2644.
- Cheng L, Hu Y, Li YY, et al. Glucagon-like peptide-1 receptor agonists and risk of bone fracture in patients with type 2 diabetes: A meta-analysis of randomised controlled trials. Diabetes Metab Res Rev. 2019;35(7):e3168.
- Montes Castillo MC, Martínez Ramírez MJ, Soriano Arroyo R, et al. Glucagon-like peptide 1 and Glucagon-like peptide 2 in relation to osteoporosis in non-diabetic postmenopausal women. Sci Rep. 2019;9(1):13651.
- Zhang L, He J, Sun X, et al. Relationship between glucagon-like peptide-1 receptor gene polymorphism and bone mineral density in postmenopausal women in Shanghai. Ann Palliat Med. 2020;9(4):1732-1741.
- Liu C, Bao X, Tian Y, Xue P, Wang Y, Li Y. Polymorphisms in the glucagon-like peptide-1 receptor gene and their interactions on the risk of osteoporosis in postmenopausal Chinese women. PLoS One. 2023;18(12):e0295451.
- Bunck MC, Eliasson B, Cornér A, et al. Exenatide treatment did not affect bone mineral density despite body weight reduction in patients with type 2 diabetes. Diabetes Obes Metab. 2011;13(4):374-7.
- Mabilleau G, Mieczkowska A, Chappard D. Use of glucagon-like peptide-1 receptor agonists and bone fractures: A meta-analysis of randomised clinical trials. J Diabetes. 2014;6(3):260-6.
- Hansen MS, Wölfel EM, Jeromdesella S. Once-weekly semaglutide versus placebo in adults with increased fracture risk: A randomised, double-blinded, two-centre, phase 2 trial. EClinicalMedicine. 2024;72:102624.
- Bollag RJ, Zhong Q, Ding KH, et al. Glucose-dependent insulinotropic peptide is an integrative hormone with osteotropic effects. Mol Cell Endocrinol. 2001;177(1-2):35-41.
- Zhong Q, Itokawa T, Sridhar S, et al. Effects of glucose-dependent insulinotropic peptide on osteoclast function. Am J Physiol Endocrinol Metab. 2007;292(2):E543-8.
- Mansur SA, Mieczkowska A, Flatt PR, Bouvard B, Chappard D, Irwin N, Mabilleau G. A new stable GIP-Oxyntomodulin hybrid peptide improved bone strength both at the organ and tissue levels in genetically-inherited type 2 diabetes mellitus. Bone. 2016;87:102-13.
- Mabilleau G, Mieczkowska A, Irwin N, et al. Beneficial effects of a N-terminally modified GIP agonist on tissue-level bone material properties. Bone. 2014;63:61-8.
- Ding KH, Shi XM, Zhong Q, et al. Impact of glucose-dependent insulinotropic peptide on age-induced bone loss. J Bone Miner Res. 2008;23(4):536-43.
- Mieczkowska A, Irwin N, Flatt PR, Chappard D, Mabilleau G. Glucose-dependent insulinotropic polypeptide (GIP) receptor deletion leads to reduced bone strength and quality. Bone. 2013;56(2):337-42.
- Westberg-Rasmussen S, Starup-Linde J, Hermansen K, et al. Differential impact of glucose administered intravenously or orally on bone turnover markers in healthy male subjects. Bone (2017) 97:261-66.
- Skov-Jeppesen K, Svane MS, Martinussen C, et al. GLP-2 and GIP exert separate effects on bone turnover: A randomised, placebo-controlled, crossover study in healthy young men. Bone. 2019;125:178-185.
- Gabe MBN, Skov-Jeppesen K, Gasbjerg LS, et al. GIP and GLP-2 together improve bone turnover in humans supporting GIPR-GLP-2R co-agonists as future osteoporosis treatment. Pharmacol Res. 2022;176:106058.
- Gasbjerg LS, Hartmann B, Christensen MB, et al. GIP’s effect on bone metabolism is reduced by the selective GIP receptor antagonist GIP(3-30)NH2. Bone. 2020;130:115079.
- Christensen MB, Lund A, Calanna S, et al. Glucose-dependent insulinotropic polypeptide (GIP) inhibits bone resorption independently of insulin and glycaemia. J Clin Endocrinol Metab. 2018;103(1):288-294.
- Torekov SS, Harsløf T, Rejnmark L, et al. A functional amino acid substitution in the glucose-dependent insulinotropic polypeptide receptor (GIPR) gene is associated with lower bone mineral density and increased fracture risk. J Clin Endocrinol Metab. 2014;99(4):E729-33.
- Kizilkaya HS, Sørensen KV, Kibsgaard CJ, et al. Loss of function glucose-dependent insulinotropic polypeptide receptor variants are associated with alterations in BMI, bone strength, and cardiovascular outcomes. Front Cell Dev Biol. 2021;9:749607.
- Styrkarsdottir U, Tragante V, Stefansdottir L, et al. Obesity variants in the GIPR gene are not associated with risk of fracture or bone mineral density. J Clin Endocrinol Metab. 2024;109(8):e1608-e1615.
- Mishra R, Raj R, Elshimy G, et al. Adverse events related to tirzepatide. J Endocr Soc. 2023;7(4):bvad016.
- Gasbjerg LS, Rosenkilde MM, Meier JJ, Holst JJ, Knop FK. The importance of glucose-dependent insulinotropic polypeptide receptor activation for the effects of tirzepatide. Diabetes Obes Metab. 2023;25(11):3079-3092.
- Pacheco-Pantoja EL, Ranganath LR, Gallagher JA, Wilson PJ, Fraser WD. Receptors and effects of gut hormones in three osteoblastic cell lines. BMC Physiol. 2011;11:12.
- Huang YM, Xu B, Kuai Z, et al. Glucagon-like peptide-2 ameliorates age-associated bone loss and gut barrier dysfunction in senescence-accelerated mouse prone 6 mice. Gerontology. 2023;69(4):428-449.
- Gobron B, Bouvard B, Legrand E, Chappard D, Mabilleau G. GLP-2 administration in ovariectomised mice enhances collagen maturity, but did not improve bone strength. Bone Rep. 2020;12:100251.
- Haderslev KV, Jeppesen PB, Hartmann B, et al. Short-term administration of glucagon-like peptide-2: Effects on bone mineral density and markers of bone turnover in short bowel patients with no colon. Scand J Gastroenterol. 2002;37(4):392-8.
- Gottschalck IB, Jeppesen PB, Hartmann B, Holst JJ, Henriksen DB. Effects of treatment with glucagon-like peptide-2 on bone resorption in colectomised patients with distal ileostomy or jejunostomy and short bowel syndrome. Scand J Gastroenterol. 2008;43(11):1304-10.
- Henriksen K, Neutzsky-Wulff AV, Bonewald LF, Karsdal MA. Local communication on and within bone controls bone remodelling. Bone. 2009;44(6):1026-33.
- Xing B, Yu J, Zhang H, Li Y. RANKL inhibition: A new target of treating diabetes mellitus? Ther Adv Endocrinol Metab. 2023;14:20420188231170754.
- Gobron B, Couchot M, Irwin N, Legrand E, Bouvard B, Mabilleau G. Development of a first-in-class unimolecular dual GIP/GLP-2 analogue, GL-0001, for the treatment of bone fragility. J Bone Miner Res. 2023;38(5):733-748.
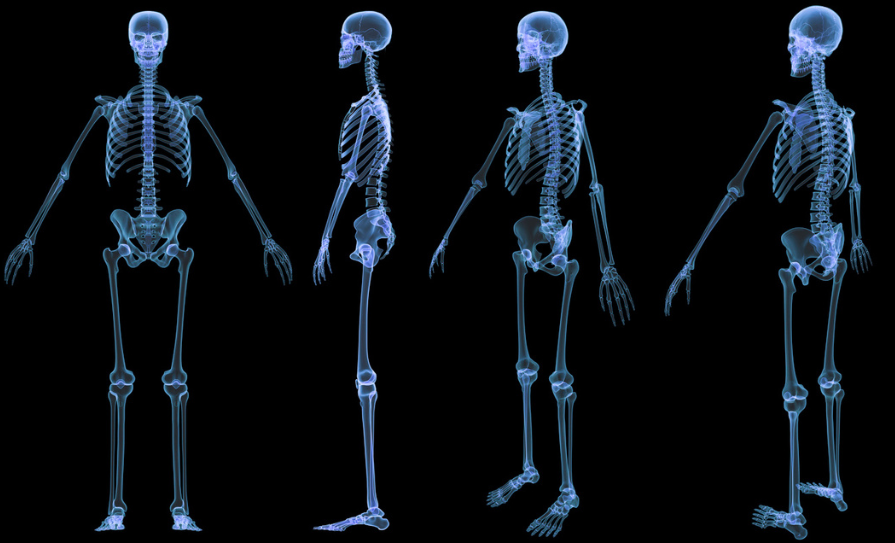